NanoFiber Technology
A Spectacular Technology Bringing Innovation to All Industries Worldwide

NanoFiber: A Spectacular Technology Bringing Innovation to All Industries Worldwide!”
NanoFiber is an exciting technology that has emerged recently and is revolutionizing many industries. This innovative material is pushing the boundaries of many industries, offering a range of applications and developments not previously thought possible.
These nanoscale fibers are known for their strength, lightness and special properties. These features of the technology enable various applications in many sectors such as healthcare, electronics, textile, construction and energy.
In the healthcare industry, the use of NanoFiber can be found in a wide range of applications, from drug delivery systems to medical devices. In the field of electronics, this technology enables the production of smaller, lighter and more powerful devices. In the textile sector, NanoFiber enables a variety of applications, from waterproof clothing to durable sports equipment.
When used in the construction industry, NanoFiber has the potential to produce durable and lightweight building materials. In the energy sector, this technology can be used to create more effective and efficient energy storage systems.
In short, NanoFiber stands out as a technology that pioneers innovation and development in different sectors all over the world by eliminating inter-industry boundaries. There is much more to be discovered in the future regarding the potential and application areas of NanoFiber.
These small strips of material are created using a variety of techniques and methods developed over hundreds of years. Around 1600, a man named William Gilbert observed how liquid behaved when affected by an electric field produced by rubbing a piece of amber. When the amber was brought close to the liquid, it formed a shape now known as the Taylor cone (see Figure 1). After this initial discovery, there were many scientists and academics who saw the potential for future applications. In 1887, British physicist Charles Vernon Boys published a manuscript about the development of nanofibers and how they might be produced in the future. Boys' findings, along with many others, probably helped American inventor John Francis Cooley apply for the first modern electrospinning patent in 1900.
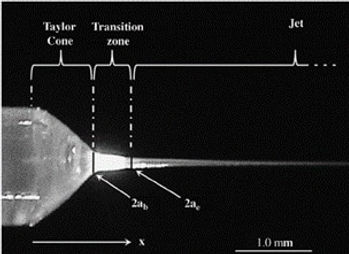
Figure 1: Taylor cone, transition zone and resulting liquid jet.
The first attempt to spin nanofibers was attempted by Anton Formhals as far back as 1934. He subsequently published the first patent providing a detailed description of the experimental procedure for nanofiber production. This led to the first patent issued in 1966 by Harold Simons for an actual machine capable of producing lightweight and thin nanofiber fabrics on a larger scale. Since then, nanofiber has become well known in the scientific community and is constantly being developed.
Nanofibers are generally around 50 to 500 nanometers in diameter, depending on the type of polymer used and the characteristics of the design. To gain some perspective, look at Figure 2 to the right. The small speck is a piece of pollen. The large strand on the left is not a nanofiber strand but a piece of human hair with a diameter of about 75,000 nm. If you look closely, you can see a network of nanofibers in the background.
There is a wide variety of polymers and compounds used to create naturally occurring polymers such as collagen, cellulose, and gelatin, as well as nanofibers such as polyurethane and lactic acid. These polymers and others are being used to develop and create a wide variety of technologies, including batteries, fuel cells, regenerative biotissue, and advanced liquid and air filtration. Although these fibers are nearly impossible to detect with the naked eye, they can cover an enormous amount of surface area relative to the overall volume of the material. Nanofiber filters are thus lightweight and breathable, making them perfect for filtering unwanted particles that can slip through traditional filter fabric.
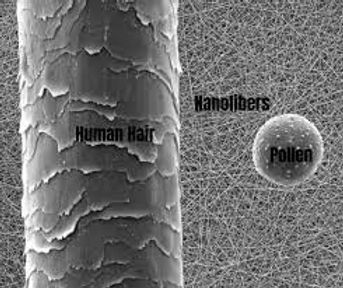
Figure 2: Size comparison of a pollen particle with a human hair. Nanofiber mesh in the background.

A thin fiber filtration layer There are superior features that come with having it. First, nanofiber filters have higher initial and ongoing efficiency compared to conventional filters. They offer low pressure losses. They are washable, washing them does not cause any wear or deformation. A nanofiber mesh can trap dust and other harmful particles much better than traditional fiber filters. They can achieve these high levels of filtration using filtering methods such as interception, diffusion, and impingement. To learn more about filtering mechanisms, please click here.
Another reason why nanofiber is superior is that filters using nanofiber layers last longer than other filters. Conventional filters reach the end of their life when the entire depth of the material is filled with debris. Nanofiber traps these particles at the surface and helps prevent them from clogging other layers of material in the filter.
We also;
We produce the nanofiber mesh with filter media consisting of a total of 3 layers of structure, protecting it between the breathable upper and lower mesh layers. Thus, the life of the filters is much longer.
Longer filter life means the user won't have to buy filters as often as before. Your energy consumption decreases. It extends the life of your systems and devices. So, although many fine fiber filters have higher upfront costs, they can help save a significant amount of money in the long run.
What is NanoFiber?
Many different methods are used to create nanofibers, such as template synthesis, melt blowing, freeze drying, and phase separation. The most commonly used technique is called electrospinning. Here, we spin our nanofiber from a base polymer. The process requires a high-voltage electric field with positive and negative charges at the ends, similar to a magnet. The polymer is loaded into an extruder at one end of the field and quickly pulled out and stretched toward the oppositely charged end, creating a long, thin strand. This results in an ultrathin network of nanofibers spun directly onto a base layer for support and support.
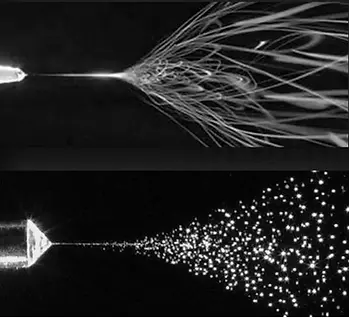
Figure 3: Electrospinning (top) and electrospray (bottom)
(source:https://www.frontiersin.org)
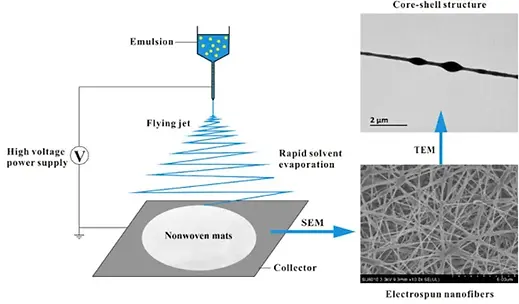
Figure 4: Electrospinning (top) and electrospray (bottom)
(source:https://www.frontiersin.org)
Figure 4 above shows how the electrospinning process is performed. Emulsion, loaded. As soon as it exits the applicator, it immediately forms a Taylor cone as a result of the high voltage power supply creating an electric field between the applicator and the collector. As it moves further into the fluid field, it becomes more unstable and turns into a spiraling, flying jet. The fluid jet then lands on a mat of material, in this case a non-woven material. The nanofiber mesh will then be used in combination with other material layers or on its own, whatever the design specifications require.
How Does Nanofiber Work?
When harmful particles such as dust, smoke, viruses and bacteria encounter the nanofiber layer, it is as if they have entered a cursed forest from which almost no one can escape. As these particles try to make their way through the dense matrix, the seemingly endless array of fibers can easily block them from passing through mechanical filtration, unlike filters that use electrostatic filtration methods.
You will often hear mask manufacturers describe their products using effectiveness and particle size. For example, N95 masks must meet a 95% filtration efficiency down to 0.3 microns to pass certification. But why 0.3 micron? Anything larger than 0.3 microns is pretty easy for filters to catch. For smaller particles, the threshold between 0.1 and 0.3 microns is the most challenging particle range for filters to capture (see Figure 5). When particles become smaller than 0.1 microns, they experience Brownian motion.
This phenomenon occurs when particles are so small that they change direction when they hit gas molecules. Brownian motion bounces particles so quickly over such a short distance that they are almost guaranteed to enter the filter fibers.
Another way to think of it is that it acts as a fishing net. The network of continuous, overlapping fibers traps larger particles and prevents them from coming out the other side. As more particles become trapped in the mesh, a type of filter cake forms throughout the material. This helps improve the overall filtration efficiency of the media over time.
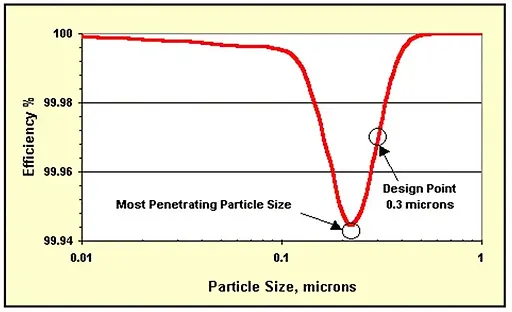
Figure 5: Typical performance of HEPA 99.97% filter.
Mechanical and Electrostatic Filtration
There are many inherent properties that distinguish nanofiber from traditional filters. In addition to being cost-effective and easily produced, nanofiber networks have high porosity and a large surface area to volume ratio. These properties make them extremely useful in applications such as protective clothing, energy storage, fiber optics, and organic tissue engineering. The main difference between nanofiber filters and other filters is the type of filtering method used.
Many filters on the market today are designed to attract and trap particles uses electrostatic forces. This may sound great at first, but they are generally not as effective as other filtration media as they are less effective at trapping larger particles such as dust and mould. Over time, they begin to lose their static charge as they trap more dust and debris. As mentioned previously, mechanical filters such as our nanofiber material tend to have higher initial efficiencies that do not decrease rapidly over time. This provides longer filter life and cleaner air output.

